Teoría de códigos y temas afines
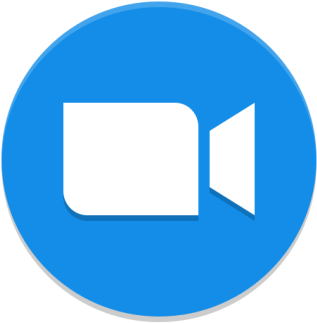
Organizadores: Ricardo Podestá (podesta@famaf.unc.edu.ar), Claudio Qureshi (cqureshi@gmail.com)
-
Monday 13
15:00 - 15:45Laticce codes
Sueli I. R. Costa (Universidade Estadual de Campinas, Brasil)
Lattices are discrete sets of the n-dimensional Euclidean space given by all integer combinations of a set of independent vectors. Lattices have being used in processes of coding for signal transmission over MIMO ( multiple input /multiple output) channels and in cryptographic schemes in the recent area of post-quantum cryptography. In this talk we give a general approach to this topic and present briefly some recent results regarding perfect lattices in different metrics, lattices constructed from codes over finite rings and an extension of the Ring-LWE problem in Cryptography which involves a more general class of algebraic lattices.
15:45 - 16:30The conorm code of an AG-code
María Chara (Universidad Nacional del Litoral, Argentina)
Let \(\mathbb{F}_q\) be a finite field with \(q\) elements. For a given trascendental element \(x\) over \(\mathbb{F}_q\), the field of fractions of the ring \(\mathbb{F}_q[x]\) is denoted as \(\mathbb{F}_q(x)\) and it is called a rational function field over \(\mathbb{F}_q\). An (algebraic) function field \(F\) of one variable over \(\mathbb{F}_q\) is a field extension \(F/\mathbb{F}_q(x)\) of finite degree. The \textit{Riemann-Roch space} associated to a divisor \(G\) of \(F\) is the vector space over \(\mathbb{F}_q\) defined as $$\mathcal{L}(G)=\{x\in F\,:\, (x)\geq G\}\cup \{0\},$$ where \((x)\) denotes the principal divisor of \(x\). It turns out that \(\mathcal{L}(G)\) is a finite dimensional vector space over \(\mathbb{F}_q\) for any divisor \(G\) of \(F\). Given disjoint divisors \(D=P_1+\cdots+P_n\) and \(G\) of \(F/\mathbb{F}_q\), where \(P_1,\ldots,P_n\) are different rational places, the \textit{algebraic geometry code} (AG-code for short) associated to \(D\) and \(G\) is defined as $$C_\mathcal{L}^F (D,G) = \{(x(P_1),\ldots, x(P_n))\,:\,x\in \mathcal{L}(G)\}\subseteq (\mathbb{F}_q)^n,$$ where \(x(P_i)\) denotes the residue class of \(x\) modulo \(P_i\) for \(i=1,\ldots,n\).
In this talk the concept of the conorm code, associated to an AG-code will be introduced. We will show some interesting properties of this new code since some well known families of codes such as repetition codes, Hermitian codes and Reed-Solomon codes can be obtained as conorm codes from other more basic codes. We will see that in some particular cases over geometric Galois extensions of function fields, the conorm code and the original code are different representations of the same algebraic geometry code.
16:45 - 17:30A Decoding Algorithm of MDS Array Codes
Cintya Wink de Oliveira Benedito (Universidade Estadual Paulista, Brasil)
This work aims to present a construction of MDS (Maximum Distance Separable) array codes constructed by using superregular matrices, in particular Vandermonde matrices and Cauchy matrices, and the Frobenius companion matrix obtained through a primitive polynomial over \(\mathbb{F}_q[x]\). Array codes are two-dimensional error correction codes whose main characteristic is the ability to correct burst of errors, that is, errors that occur in consecutive bits. MDS codes are codes in which the minimum distance is the maximum possible. This characteristic is important because, in coding theory, the minimum distance is related to the error correction capacity of the code in addition to providing maximum protection against failures of a device for a given amount of redundancy. Based on this construction, a decoding algorithm is presented to correct up to two bursts of errors in MDS array codes with parameters \([m + k, k, m + 1]\) over \(\mathbb{F}_q^b\), for all \(m \geq 4\), where \(b\) refers to the length of the error. In addition, some examples are presented to correct three bursts of errors. This is a joint work with Débora Beatriz Claro Zanitti, São Paulo State University (UNESP).
17:30 - 18:15AG codes, bases of Riemann-Roch spaces and Weierstrass semigroups
Horacio Navarro (Universidad del Valle, Colombia)
In this talk we are going to discuss some results on the explicit construction of bases of Riemann-Roch spaces, weierstrass semigroups and the floor of certain divisors. We also will apply these results to get AG codes with good parameters.
-
Tuesday 14
15:00 - 15:45The weight distribution of irreducible cyclic codes associated with decomposable generalized Paley graphs
Denis Videla (Universidad Nacional de Córdoba, Argentina)
We use known characterizations of generalized Paley graphs which are Cartesian decomposable to explicitly compute the spectra of the corresponding associated irreducible cyclic codes. As applications, we give reduction formulas for the number of rational points in Artin-Schreier curves defined over extension fields. This talk is based on a recent joint work with Ricardo Podestá.
15:45 - 16:30Direct sum of Barnes-Wall lattices via totally real number fields
Grasiele C. Jorge (Universidade Federal de São Paulo, Brasil)
Let \(\mathbb{K}\) be a number field of degree \(n\), \(\mathcal O_{\mathbb{K}}\) its ring of integers and \(\alpha \in \mathcal O_{\mathbb{K}}\) a totally real and totally positive element. In 1999, Eva Bayer-Fluckiger introduced a twisted embedding \(\sigma_{\alpha}:\mathbb{K} \rightarrow \mathbb{R}^{n}\) such that if \(\mathcal I \subseteq \mathcal O_{\mathbb{K}}\) is a free \(\mathbb{Z}\)-module of rank \(n\), then\(\sigma_{\alpha}(\mathcal I)\) is a lattice in \(\mathbb{R}^{n}\). It was shown that if \(\mathbb{K}\) is a totally real number field, then \(\sigma_{\alpha}(\mathcal I)\) is a full diversity lattice. In this talk we will approach constructions of direct sum of Barnes-Wall lattices \(BW_n\) for \(n=4,8\) and \(16\) via ideals of the ring of the integers \(\mathbb{Z}[\zeta_{2^{r}q} + \zeta_{2^{r}q}^{-1}]\) for \(q = 3, 5\) and \(15\). Our focus is on totally real number fields since the associated lattices have full diversity and then may be suitable for signal transmission over both Gaussian and Rayleigh fading channels. The minimum product distances of such constructions are also presented here. This is a joint work with Jo\~{a}o E. Strapasson, Agnaldo J. Ferrari and Sueli I. R. Costa and it was partially supported by Fapesp 2013/25977-7, 2014/14449-2 and 2015/17167-0 and CNPq 432735/2016-0 and 429346/2018-2.
16:45 - 17:30The Generalized Covering Radii of Codes
Marcelo Firer (Universidade Estadual de Campinas, Brasil)
The generalized covering-radius hierarchy of a linear code is a new property of codes which was motivated by an application to database linear querying, such as private information-retrieval protocols. It characterizes the trade-off between storage amount, latency, and access complexity, in such database systems.
In this work, we introduce and discuss three definitions for the generalized covering radius of a code, highlighting the combinatorial, geometric, and algebraic properties of this concept and prove them to be equivalent. We also discuss a connection between the generalized covering radii and the generalized Hamming weights of codes by showing that the latter is in fact a packing problem with some rank relaxation.
Other contributions to the subject include bounds relating various parameters of codes to the generalized covering radii and an asymptotic upper bound (for particular parameters) which shows that the generalized covering radii improves the naive approach.
Joint work with Dor Elimelech and Moshe Schwartz (Ben-Gurion University/Israel).
17:30 - 18:15A novel version of group codes
Ismael Gutiérrez (Universidad del Norte, Colombia)
Let \(G\) be a finite abelian group, with \(G=\prod_{i=1}^n \langle g_{i} \rangle \) where \(|\langle g_{i} \rangle|=m_{i}\). Then every element in \(G\) can be uniquely written as \(\prod_{i=1}^n g_i^{\epsilon_i}\) where \(0\leq \epsilon_i \leq m_{i}-1 \). To determine a measure of the separation between two elements of \(G\) we use the \texttt{Minkowski distance \(l_1\)}, which is given by $$l_{1}\Big(\prod_{i=1}^n g_i^{\epsilon_i}, \prod_{i=1}^n g_i^{\delta_i}\Big) = \sum_{i=1}^n |\epsilon_i-\delta_i|.$$
A grid code \(\mathscr{C}\) is a subset of \(G\), if \(\mathscr{C}\) is subgroup of \(G\), then it is said that \(\mathscr{C}\) is a group code. The elements of \(\mathscr{C}\) are called codewords. The minimum distance \(d\) of a code \(\mathscr{C}\) is defined as usually, that is, as the smallest distance between any two different elements of \(\mathscr{C}\). Let \(\mathscr{C}\) be a code of \(G\) with minimum distance \(d\). Then we say that \(\mathscr{C}\) is a \((n,|\mathscr{C}|,d)\)-code over \(G\) and \((n,|\mathscr{C}|,d)\) are its parameters.
In this talk, we consider such codes, and we prove some classical results on block codes, like Singleton Bound and others.